L44A
Ryerson Woods, Lake Cnty Forest Preserve, IL, USA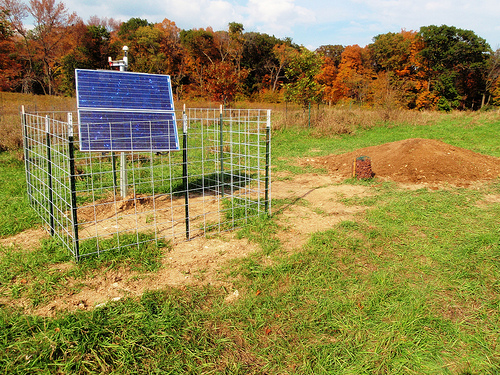
The USArray component of the NSF-funded EarthScope project ended its observational period in September 2021 and all remaining close-out tasks concluded in March 2022. Hundreds of seismic stations were transferred to other operators and continue to collect scientific observations. This USArray.org website is now in an archival state and will no longer be updated. To learn more about this project and the science it continues to enable, please view publications here: http://usarray.org/researchers/pubs and citations of the Transportable Array network DOI 10.7914/SN/TA.
To further advance geophysics support for the geophysics community, UNAVCO and IRIS are merging. The merged organization will be called EarthScope Consortium. As our science becomes more convergent, there is benefit to examining how we can support research and education as a single organization to conduct and advance cutting-edge geophysics. See our Joining Forces website for more information. The site earthscope.org will soon host the new EarthScope Consortium website.
By Maia ten Brink
On February 15, 2013, an asteroid 18 meters wide plummeted into Earth’s atmosphere and exploded over Chelyabinsk, Russia, with the force of 500 kilotons of TNT. Nearby eyewitnesses saw an intense light flare across the gray morning sky, leaving a trail of smoke. The meteor’s explosion generated low-frequency shock waves, known as infrasound, so powerful that, on the ground 30 kilometers below the explosion, thousands of windows blew out, more than 7,000 buildings sustained damage, and nearly 1,500 people were injured. These infrasound waves traveled twice around the world and were recorded by the USArray Transportable Array, a seismic network spanning the United States, as they bounced off Earth’s surface and shook the ground.
For Michael Hedlin, a geophysicist at the Scripps Institute of Oceanography in San Diego, the Chelyabinsk meteor was a once-in-a-lifetime sonic experiment. Hedlin has been investigating atmospheric acoustics since the late 1990s. He uses data from the Transportable Array (TA), originally intended to record seismic waves in the solid Earth, to learn how infrasound waves lower than 20 Hertz propagate through the atmosphere.
“There are all these sound waves traveling through the atmosphere, traveling past us at any moment wherever we are,” says Hedlin. “And people have no idea that they’re there because they are such a low frequency sound that they can’t hear them or even feel them.” At close range and large amplitudes, however, infrasound waves can make a physical impact. Hedlin had the opportunity to stand aboard a Kitty Hawk aircraft carrier as it was launching fighter planes off using a catapult. He says he felt pulses of sound vibrating within his chest cavity.
Although infrasound is below humans’ hearing range, elephants, whales, and other animals actually use it to communicate across immense distances. To their ears, the Chelyabinsk meteor must have created a terrible racket.
“The planet is really noisy. It is always making these natural sounds that we can listen to and learn more about how the planet functions,” says Hedlin.
Hedlin has an extremely deep voice, fitting for someone who studies low-frequency acoustics. He’s a lanky Californian who wears skinny jeans and flip-flops to work, exercises avidly, and keeps a kayak in his wood-paneled office on the cliffs of La Jolla overlooking the Pacific Ocean. His wife and collaborator, Catherine de Groot-Hedlin, has a matching kayak in her office, which is just around the corner. They keep framed photos of each other propped on their respective desks from their graduate student days at the University of California, San Diego.
Hedlin and de Groot-Hedlin first started looking for infrasound waves in the TA seismic data in 2007 after NASA’s Atlantis space shuttle made an emergency landing at Edwards Air Force Base north of Los Angeles. The TA’s seismic sensors, placed along the West Coast at the time, picked up infrasound waves from the shuttle’s re-entry. De Groot-Hedlin remembers hearing the windows shake as the shuttle flew overhead. Since then, Hedlin says, seismic readings from the TA have provided “an acoustic fingerprint of the United States.”
The sound sources Hedlin and de Groot-Hedlin usually pick up are on a smaller scale: bombs, mining explosions, sonic booms, landslides, volcanic eruptions, and large storms. “Basically anything natural or man-made that moves a lot of air,” Hedlin explains.
Some of the largest man-made infrasound signals come from nuclear bombs. Part of Hedlin’s work involves monitoring the skies to ensure that nations uphold the Comprehensive Nuclear-Test-Ban Treaty, an international agreement to abstain from nuclear testing. Events like the Chelyabinsk meteor provide a good approximation for small nuclear explosions1. Right now, Hedlin says, the only nuclear detonations they detect occur underground in North Korea.
Infrasound may also be able to provide early warnings about avalanches and volcanic eruptions. “A volcano is a very turbulent, hot location,” says Hedlin. “It will move the ground, generate seismic waves, and can also send pulses [of infrasound] into the atmosphere.” Volcanic ash poses a threat for airplanes flying nearby, and infrasound waves can be powerful enough to cause damage several kilometers downwind.
But before infrasound can be used for real-time hazard detection, it needs to be better understood. “You can determine that you have an infrasound source, but then you have the mystery of what caused it,” Hedlin says. Acoustic scientists don’t want to sound a false alarm about a nuclear explosion; it could have dire political consequences. “That’s why we’re doing this research to try to understand why the atmosphere is stretching signals,” he says. “We want to learn how to model the [infrasound] propagation well enough to understand what happened at the source.”
In 2011, Hedlin and his colleague Frank Vernon got a proposal funded to add infrasound sensors to all 400 TA seismic stations. Each TA station is now equipped with an infrasound microphone and two pressure sensors that measure air pressure at 40 samples per second, as well as a broadband seismic sensor to detect ground motion. “The beautiful thing about this project was that the Transportable Array infrastructure was already in place,” Hedlin says. Now the TA is the world’s most extensive infrasound array, providing Hedlin and other researchers with an unprecedented range of atmospheric and meteorological data.
Screen shot from April 2011 when a two- to six-hour gravity wave
propagated slowly (about 40 meters per second) from south to north across
theTransportable Array. Red is high atmospheric pressure, blue is low.
The scale is in Pascals. The red squares are tornadoes.
With seismic stations every 70 kilometers, it’s easy to track signals from one station to the next across the TA network. Compared to the International Monitoring System, a sparse global infrasonic array with stations 2,000 kilometers apart, it is easier to study infrasound in greater detail. “The Transportable Array is really the backbone of our research right now,” says Hedlin. “It’s been incredibly helpful to us.”
Hedlin has used TA findings to model how infrasound waves propagate through the atmosphere. Just like seismologists use seismic waves from earthquakes to image the layers inside Earth, Hedlin looks at how infrasound waves stretch and warp as they travel in order to get an accurate picture of the atmosphere. But while Earth’s structure changes extremely slowly, the atmosphere is constantly changing.
Infrasound waves travel extremely slowly, at 300–310 meters per second. That’s less than 10% of the speed of seismic waves. What starts as a relatively simple sound signal from an explosion gets modified as it ripples out for hundreds or thousands of kilometers. Turbulence, seasonal winds, and gravity waves—sound waves so slow that gravity acts on them—alter infrasound. “Everything in the atmosphere from the temperature to the direction and speed of the wind is important to determine how infrasound propagates,” says Hedlin.
Meanwhile, de Groot-Hedlin has been scouring the TA data for even lower-frequency waves known as gravity waves. “A gravity wave is a bundle of air like a tsunami,” she explains. “With a tsunami, water gets displaced from its usual position. It gets uplifted [by fault movements producing an earthquake] and it wants to spread out and down.” In the atmosphere, convective events caused by rising columns of warm air, severe storms, or wind flow over mountains might set off long-period gravity waves.
Sometimes, analyzing the entire array can overlook activity happening on a more local scale, so de Groot-Hedlin had the idea to break down the TA’s 400 stations into triangular sub-arrays consisting of three adjacent stations. She searched “Tornado Alley” in the central United States for gravity waves caused by swarms of tornadoes and passing over each sub-array, which she called triads.
De Groot-Hedlin came to the field of atmospheric acoustics from hydro-acoustics, the science of sound moving through water. Now that she has created a code that automatically detects gravity waves, she has to learn more about atmospheric science to figure out how gravity waves interact with climate and weather dynamics.
For Hedlin, studying the atmosphere was a logical extension of his graduate school seismology research on Earth’s interior. “Earth is a system. You’ve got the solid interior, the atmosphere, the cryosphere (the ice caps), the hydrosphere (the ocean), and all these parts are interacting and communicating,” he says. For example, when an earthquake occurs under a mountain range, Hedlin says, “it will cause the mountains to sway back and forth really slowly. As they rock, they push on the air in the atmosphere and generate low-frequency sound waves.” Even extra-terrestrial objects like the Chelyabinsk meteor can affect Earth’s systems.
Barring further surprise meteors or nuclear explosions, Hedlin and de Groot-Hedlin are looking forward to the TA’s upcoming deployment in Alaska. “There are phenomena in Alaska that we don’t have here in the continental US. There are a lot of volcanic eruptions along the Aleutians for example,” says Hedlin. Aurora, frequent landslides, and calving glaciers also generate infrasound waves.
“Much of what we are going to learn is still in the future,” Hedlin says. “We have been collecting the data for a relatively short time. We are just scratching the surface of what is in this huge data set.”
_______________
1 The Tsar Bomba, the largest nuclear test ever conducted, occurred in October 1961 over Novaya Zemlya, Russia. One hundred times as powerful as the Chelyabinsk meteor explosion, it had an explosive yield of 50–58 megatons of TNT.